Continuing on with right heart failure with part 3 of the 4-part series. The next core component to discuss is afterload. I believe that afterload is the most important hemodynamics variable to look at in RV failure. When I am trying to optimize a patient’s right ventricle I always make sure I have optimized the afterload before moving on to the other components. If you have not read Management of acute right ventricular failure in the intensive care unit (1) I recommend reading it, especially before part 4.
Breaking down cardiac output in terms of the right heart.
Let’s relook at the breakdown of cardiac output into its four core components (Figure 1). I have added in the preload and contractility components that were filled out in parts 1 and 2.
Figure 1: Cardiac output breakdown
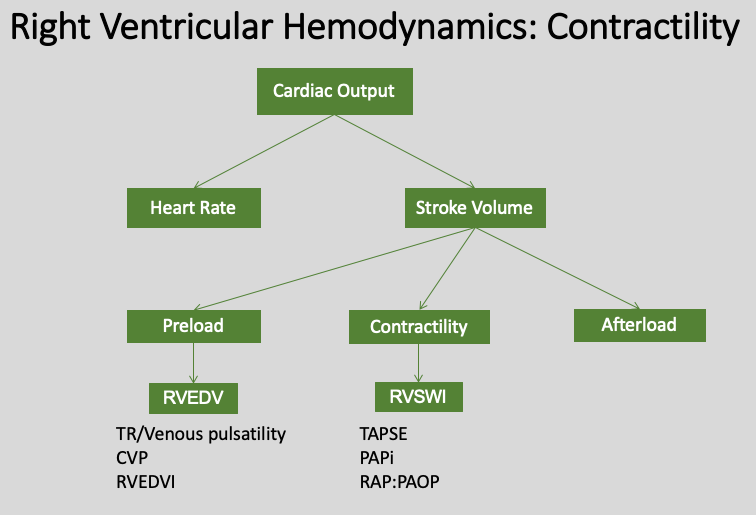
3. Afterload
Definition:
Afterload is defined as the resistance the heart must overcome during systole. This is the simplified definition, the more complete definition is the wall stress that occurs during systole. Wall stress (T) is defined using LaPlace’s law which is the pressure in the ventricle during systole (P) multiplied by the wall radius (r) of the ventricle divided by double the wall thickness (H) (2).
T = (P x r)/2H
This means that afterload is directly proportional to the pressure and radius of the ventricle, and inversely proportional to the wall thickness.
I visualize afterload as a wall that is raised in the pulmonary artery that makes it harder to get blood from the right heart to the left heart.
Disease physiology:
The right ventricle is more sensitive than the left ventricle to afterload. With small changes in afterload, there is a significant drop in stroke volume in the right ventricle compared to the left ventricle (Figure 2).
Figure 2: Ventricles response to afterload (1)
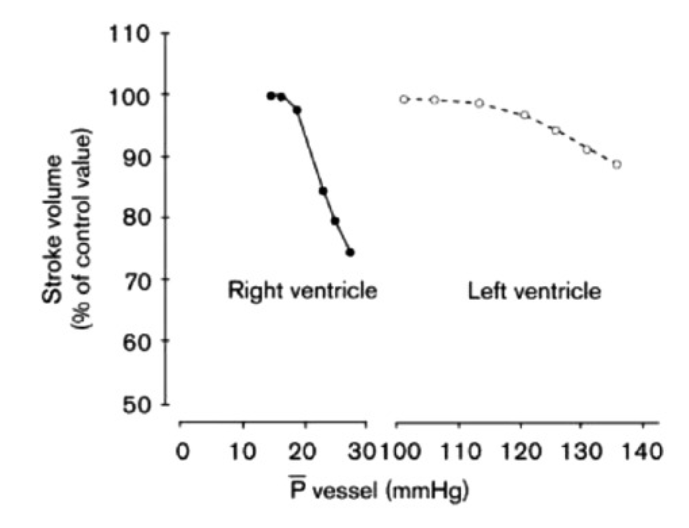
This decrease in stroke volume leads to an increased volume at the end of systole and diastole. The increased preload causes ventricular dilation and tricuspid valve separation leading to tricuspid regurgitation and reversal of flow. The increased preload also leads to decreased contractility as it moves the ventricle to the descending limb of the Starling curve. Additionally, the increased pressure and volume will compress small arterioles and capillaries in the thin wall of the ventricle and lead to ischemia. The ischemia leads to worsening contractility which further worsens stroke volume. This also causes decreased preload to the left ventricular causing low cardiac output. Low cardiac output decreases right coronary perfusion which also leads to worsening RV function and goes into a vicious spiral leading to death.
Figure 3: RV Failure Death Spiral (3)

Measuring Afterload:
Estimated PASP:
The pulmonary artery systolic pressure (PASP) can be estimated non-invasively. This allows a quick, reliable evaluation of pulmonary pressures. As mentioned above, the pulmonary pressures increase and the RV dilates creating tricuspid regurgitation. During systole, the higher the pulmonary pressures, the more afterload to push against, and the higher the velocity of the blood going backward through the tricuspid valve. The velocity of the TR can be used to estimate the right ventricular systolic pressure (RVSP) which equals the pulmonary artery systolic pressure (PASP).
The ability to estimate PASP is based on Bernoulli’s equation and the conservation of energy between the speed and pressure of a fluid.
Bernoulli’s Equation:

This equation is simplified after applying it to the circulatory system and is beautifully explained in this YouTube video: https://www.youtube.com/watch?v=cO6B9Q3At1Y
Figure 4: Simplified Bernoulli’s Equation:


Tricuspid regurgitation velocity (TRV) is measured with TTE in either the apical-4 view, parasternal short-base view, or the RV inflow view. The apical-4 is the most common view used, but it is good to be able to look at multiple views if one is unable to be obtained. Continuous-wave Doppler (CW) is used through the tricuspid valve and a TR max velocity is measured. It is typically measured in cm/s and will need to be converted to m/s for the equation (Figure 5). This value is added to the estimated or measured CVP. Estimated CVP is based on the IVC and its size and variability. Any estimated PASP value > 40mmHg is considered elevated and infers that there is pulmonary hypertension (4,5).
Figure 5: Tricuspid Regurgitation Max Velocity
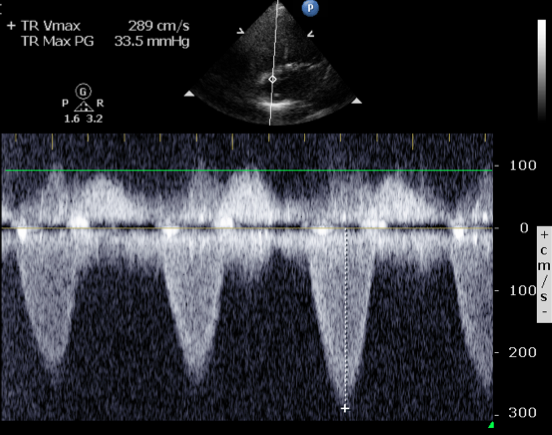
Table 1: Estimating CVP using IVC on TTE
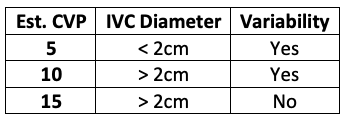
mPAP:
The mPAP is measured invasively using a pulmonary artery catheter. mPAP is the standard used to diagnosed pulmonary hypertension. Updated 2018 guidelines have lowered the value required to diagnose pulmonary hypertension from ≥25 mmHg to ≥20 mmHg. mPAP can be used in the acute setting in the ICU, but with pulmonary artery disease, the mPAP will increase or decrease with changes in MAP making its absolute value less useful. A mPAP >20 mmHg can be used to look closer at RV afterload as a cause for hemodynamic issues.
Figure 6: Pulmonary Artery Pressures on the Monitor

mPAP:MAP:
The mPAP to MAP ratio is a much better marker for afterload issues than mPAP alone. It has been used to look at the outcomes for advanced heart failure, pulmonary arterial hypertension, and post-LVAD. Normally the mPAP should be ¼ the MAP. The higher the ratio the worse the pulmonary hypertension. Studies have shown increased complications and worsened mortality with as low as a 1/3 ratio (5-7).
PVR:
Pulmonary vascular resistance (PVR) is the standard for evaluating RV afterload. It is the actual measure of the resistance the RV must overcome during systole. PVR is left in Wood Units whereas SVR is changed to dynes. As with any equation looking at resistance, it is the change in pressure across the system divided by flow.
PVR = (mPAP – LAP)/CO
The reason that PVR is a good marker is that pulmonary pressures can be elevated due to left heart failure, but the actual resistance to flow will not be elevated. This means that the issue is not due to the pulmonary artery system or lung, that it is just back pressure from the left heart dysfunction. If the left heart can be optimized then the pulmonary pressures should improve. Over time left heart disease can cause pulmonary hypertension with an elevated PVR.
Differentiating precapillary and postcapillary:
It is important for treatment to figure out if the pulmonary hypertension is precapillary or postcapillary. For this differentiation, the capillaries, which is where lung causes are involved, are considered precapillary along with everything proximal to the capillaries. Postcapillary is due to the left heart. If it is precapillary then pulmonary vasodilators have a role in the treatment. If it is due to left heart disease pulmonary vasodilators can be detrimental. As discussed in part 1: preload, the LV is thick and does not handle preload as well as the RV. When pulmonary vasodilators are given it increases preload to the LV, and if there is LV dysfunction, the increased preload can worsen hemodynamics, increase pulmonary edema, and lead to failure. In order to differentiate between precapillary and postcapillary, the PVR and pulmonary artery occlusion pressure (LAP), are obtained.
Table 2: Differentiating Precapillary and Postcapillary
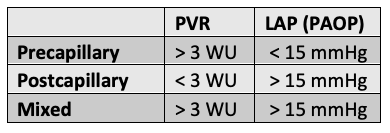
For a Deeper Look
ECU Angle:
The worse the pulmonary hypertension, the worse the RV dilation. This dilation will increase the angle of the apex of the RV. That angle has been found to equate to the PASP. A line is drawn through the septum and another along the epicardium of the RV apex. The degree of the angle is the PASP and is termed the External Chamber Union (ECU) angle (8).
Figure 7: External Chamber Union Angle and PASP

Potential Pitfalls:
Looking at the pulmonary pressures can be misleading in acute obstruction with pulmonary hypertension. Acute submassive/massive pulmonary embolism is the best example of this. When there is an acute blockage that creates significant afterload or resistance to the forward flow of blood during systole, it can overwhelm the RV and the RV cannot mount pressure. The RV free wall can actually become akinetic in large PEs and is called McConnell’s sign. In these situations, a normal pulmonary artery pressure does not tell the whole story and can be misleading as to the extent of the RV dysfunction. This increase in pressure can cause flattening of the interventricular septum during systole, also known as a D-sign. This is seen in acute PE and can help guide management. When there is flattening during diastole it is due to volume overload, as discussed in part 1, but during systole, it is pressure overload. This finding should signify that there is too much RV pressure even if the PA pressures are not elevated.
Figure 8: D-sign during systole:
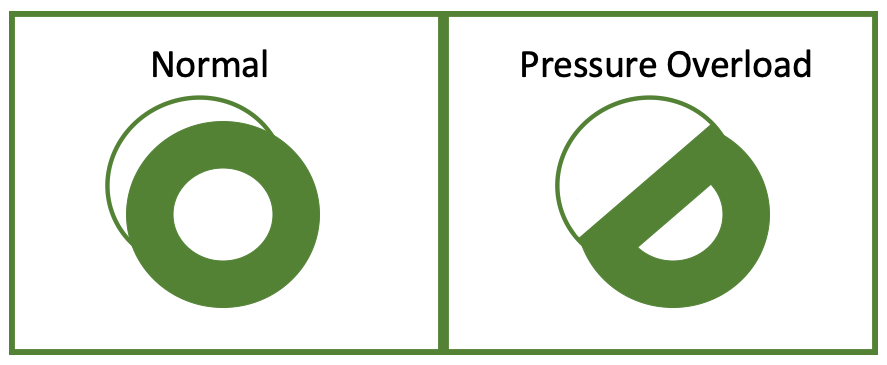
Summary:
Increased afterload is the most common cause of RV dysfunction in the ICU and can lead to contractility and preload issues. When it is not addressed the RV shock spiral starts. It should be the first component of RV failure that should be evaluated and optimized. This will save a lot of time, effort, and resources. If increased afterload is leading to decreased contractility, then treating the afterload will improve the contractility, and an RV support device can be avoided.
I try to look with point-of-care ultrasound (POCUS) or look at the official TTE for the TR max velocity and any signs of septal flattening. The septum may also have a shutter or bounce to it with respiration that signifies changes in pressure with intrathoracic pressure. I will make sure I look at the septum and TR through a couple of breathing cycles to assess for any changes. In severe cases, I will place a pulmonary artery catheter (PAC) and make sure the mPAP:MAP ratio is less than ½ as an initial goal.
Figure 9: RV Afterload

Figure 10: RV Afterload Measurements
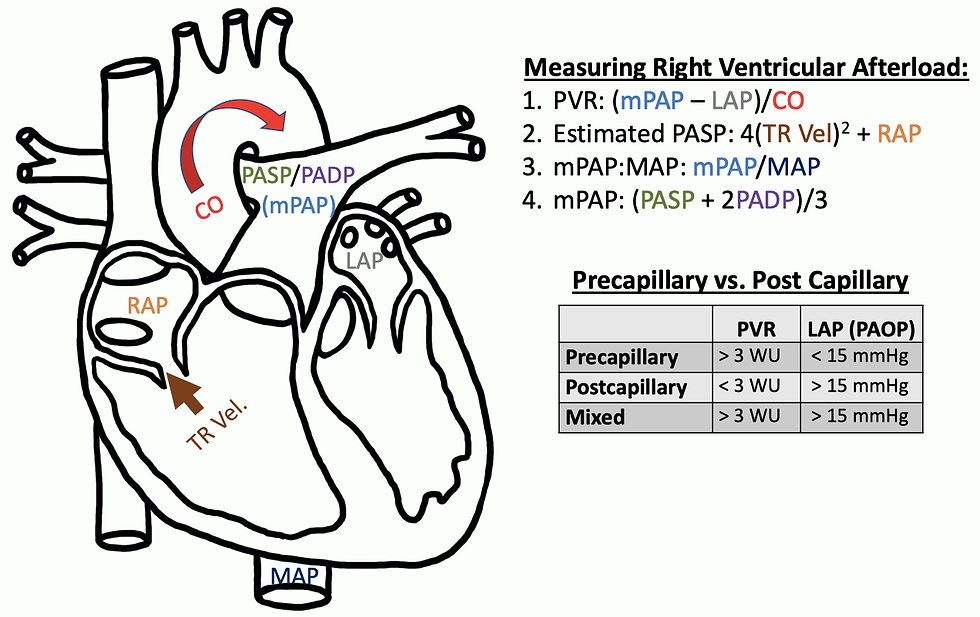
Table 3: Afterload Assessment
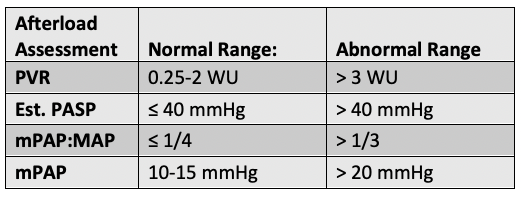
References:
1. Ventetuolo CE, Klinger JR. Management of acute right ventricular failure in the intensive care unit. Ann Am Thorac Soc. 2014;11(5):811-822. doi:10.1513/AnnalsATS.201312-446FR
2. Tedford RJ. Determinants of right ventricular afterload (2013 Grover Conference series). Pulm Circ. 2014;4(2):211-219. doi:10.1086/676020
3. Konstantinides SV, Meyer G, Becattini C, et al. 2019 ESC Guidelines for the diagnosis and management of acute pulmonary embolism developed in collaboration with the European Respiratory Society (ERS): The Task Force for the diagnosis and management of acute pulmonary embolism of the European Society of Cardiology (ESC). Eur Respir J. 2019;54(3):1901647. Published 2019 Oct 9. doi:10.1183/13993003.01647-2019
4. Rudski LG, Lai WW, Afilalo J, et al. Guidelines for the echocardiographic assessment of the right heart in adults: a report from the American Society of Echocardiography endorsed by the European Association of Echocardiography, a registered branch of the European Society of Cardiology, and the Canadian Society of Echocardiography. J Am Soc Echocardiogr. 2010;23(7):685-788. doi:10.1016/j.echo.2010.05.010
5. Nguyen A, Deschamps A, Varin F, Perrault L, Denault A. A Pathophysiological Approach to Understanding Pulmonary Hypertension n Cardiac Surgery. Perioperative Considerations in Cardiac Surgery. 2012. 277-306.
6. Robitaille A, Denault AY, Couture P, et al. Importance of relative pulmonary hypertension in cardiac surgery: the mean systemic-to-pulmonary artery pressure ratio. J Cardiothorac Vasc Anesth. 2006;20(3):331-339. doi:10.1053/j.jvca.2005.11.018
7. Mazimba S, Schubert S, Solanki J, Mwansa H, et al. Abstract 20172: Increased Pulmonary-Systemic Pressure Ratio is Associated with Adverse Events in Advanced Heart Failure. Circulation. 2017;136:A20172.
8. Sanchez H, Buch A, Suggs J, Thomassen M, et al. Angle Between the Right Ventricular Free Wall and the Interventricular Septum at the Apex as a Marker of Pulmonary Hypertension. Am J Resp Crit Care Med. 2019;199:A6813
Comments