Part I: Constructing the equation of motion
It takes a certain amount of pressure to get air into the lungs and ventilate them. The pressure will vary depending on the patient and their illness. By looking at the airway in its individual components you can create the equation of motion. The equation of motion is like the oxygen delivery equation for resuscitation, it is the equation that unifies all the components of breathing and mechanical ventilation.
The equation of motion is a variation of the transrespiratory pressure. The transrespiratory pressure is the pressure gradient that allows gas flow in and out of the lungs.
The equation of motion can look intimidating, but breaking it down and creating it from its individual components, it is easy to see how intrinsic and extrinsic forces affect the ability to ventilate.
As discussed in a previous blog, the airway can be divided into the conductive airway and the respiratory airway. The conductive airway brings air to the respiratory airway but does not participate in ventilation. The respiratory airway is where gas exchange takes place. The conductive airway is the anatomical dead space and the respiratory airway is the alveolar volume that when multiplied by the respiratory rate creates alveolar minute ventilation.
Figure 1:
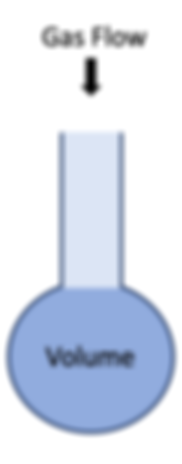
1. Conductive Airway
The equation of motion starts with the conductive airway which causes resistance to the gas getting to the alveoli. The pressure needed to overcome this resistance is known as the transairway pressure.
Using a variation of Ohm’s law, V = IR, for fluid and gas resistance equals the change in pressure divided by the flow.
Resistance = △Pressure/Flow
Using this equation, resistance is directly proportional to pressure and inversely proportional to flow. Solving for flow, F = △P/R, shows an increase in the pressure change would lead to higher flow, while an increase in resistance leads to lower flow.
For the equation of motion, we are looking at pressure and rearranging to solve for pressure:
Pressure = Resistance x Flow
Figure 2:

Transairway Pressure = Airway Resistance x Gas Flow
In a mechanically ventilated patient, there are also artificial airways along with the conductance airways that create resistance and need to be overcome as well.
2. Respiratory Airway
The second part of the equation is the pressure needed to expand the alveoli to allow air in for gas exchange. This pressure is known as the transthoracic pressure.
The ease of the ability to expand the alveoli depends on the compliance of the alveoli. Compliance is how much the pressure changes with a change in volume.
Compliance = △Volume/△Pressure
If a large amount of volume can be placed with only a small change in pressure then there is a high compliance. If there is a large increase in pressure there is a lower compliance. Then rearrange the equation to solve for pressure
Pressure = Volume/Compliance
Figure 3:

Transthoracic Pressure = Tidal Volume/Compliance
Definitions (1):

Putting all this together, the transrespiratory pressure is the transairway pressure plus transthoracic pressure.
Transrespiratory Pressure = Transairway Pressure + Transthoracic Pressure
Figure 4:

Formulas:

Now, with some quick substitutions for the definitions:
Transrespiratory Pressure = Transairway Pressure + Transthoracic Pressure
TRP = TAP + TTP
TAP = R x F
TTP = V/C
↓
TRP = R x F + V/C
Transrespiratory pressure is a gradient between the sum of the pressure generated by the respiratory muscles (Pmusc) along with the pressure generated by the ventilator (Pvent) minus the alveolar pressure (Palv) or the PEEP.
TRP= (Pmusc + Pvent) – PEEP
The final equation with all substitutions:
Pmusc + Pvent = R x F + V/C + PEEP
Or, if you prefer elastance over compliance (E = 1/C):
Pmusc + Pvent = R x F + V x E + PEEP
Hopefully, it is easy to see that this equation is still just two parts. The total pressure needed to get gas into the lung requires a resistive pressure plus a distending pressure.
Total Pressure = Resistive Pressure + Distending Pressure
Part II: Breaking down the equation of motion
Breaking down the equation of motion can narrow down our clinical differential and help make a better decision at the bedside.
Pulmonary diseases with elevated pulmonary pressures can be divided into different categories based on the equation of motion. The patient can have a resistance problem where the resistance is too high causes the pressure to increase or the patient can have a compliance problem where the compliance is too low causing the pressure to increase.
Table 1: Pulmonary diseases causing equation of motion abnormalities

To differentiate between these two, the alveolar compliance and airway resistance need to be calculated.
*The alveolar compliance is the easier of the two to calculate. This is done in a volume mode with a constant square flow pattern since the flow will be constant and pressure will be ascending as shown in the pressure scalar (Figure 5).
Figure 5: Pressure Scalar

Calculating alveolar compliance
If we do an inspiratory hold, it brings the flow to zero. This removes resistance from the equation.
Pmusc + Pvent = R x F + V/C + PEEP
↓
Pmusc + Pvent = R x (0) + V/C + PEEP
↓
Pmusc + Pvent = V/C + PEEP
The pressure becomes the plateau pressure (Pplat), the volume is the tidal volume (TV), the compliance becomes the static compliance (Cst), since it is a respiratory hold and the airway is static, and the PEEP is unchanged.
New equation:
Pplat = TV/Cst + PEEP
The equation can be solved for static compliance:
Compliance of the alveoli:
Cst = TV/(Pplat – PEEP)
Looking at the pressure scalar, the pressure required to overcome the compliance is shown. This pressure required over time is the elastic work. Elastic work is the work done to overcome the elastic recoil of the lung and chest (Figure 6).
Figure 6:

Low Compliance Differential:
Disease processes that decrease compliance lead to increased pressures or decreased volumes. In volume control mode, the pressure will increase due to an increase in plateau pressure (Pplat) and will require increased work (Figure 7).
Figure 7:

Table 2: Low compliance diseases
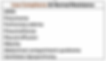
If we paralyze the patient, it will leave just the ventilator pressure (Pvent) and remove ventilator dyssynchrony from the differential.
Calculating airway resistance:
Looking at the total pressure or peak inspiratory pressure (PIP) and then removing the plateau pressure, the remaining pressure can be used to calculate the airway resistance.
Pmusc + Pvent = R x F + V/C + PEEP
Using the previous equation:
Pplat = V/C + PEEP
and substitute Pplat into the equation of motion and substitute Pmusc + Pvent with PIP
New equation:
PIP = R x F + Pplat
and then solve for resistance since flow is known and constant and PIP and Pplat will be known:
Resistance of the airway:
R = (PIP – Pplat)/F
Using the pressure scalar, the resistance can be seen after removing the pressure for alveolar inflation. This pressure required over time is the resistive work. Resistive work is the work to overcome airway and tissue resistance (Figure 8).
Figure 8:

High Resistance Differential:
Disease processes that increase the resistance lead to increased pressures or decreased volumes. In volume control mode, the pressure will increase due to an increase in total pressure or peak pressure (PIP) without an increase in plateau pressure (Pplat). This will require increased resistive work (Figure 9).
Figure 9:

Table 3: High resistance disease processes

Looking at elevated pulmonary pressures another way:
The most commonly taught way to differentiate between these two processes is by looking at the difference between peak inspiratory pressure (PIP) and plateau pressure (Pplat). If the PIP and Pplat are both elevated, it is a compliance issue. If the PIP is elevated and the Pplat is normal, it is a resistance issue. The resistance and compliance do not have to be calculated to differentiate them, you just need an inspiratory hold. But the equation of motion is helpful to understand how the diseases lead to elevated pressures.
Other calculations:
Calculating dynamic compliance:
If we look at the pressure at the end of inspiration without doing an inspiratory hold, it is the peak inspiratory pressure at zero flow and so resistance is removed.
Pmusc + Pvent = R x F + V/C + PEEP
↓
Pmusc + Pvent = R x (0) + V/C + PEEP
↓
Pmusc + Pvent = V/C + PEEP
Since there is no inspiratory hold, it is the compliance of the whole respiratory system.
New equation:
PIP = TV/Cdyn + PEEP
The compliance is the dynamic compliance, for the whole respiratory system, and the volume is TV again.
Solving for compliance, the new equation:
Cdyn = TV/(PIP – PEEP)
Table 4: Normal compliance and resistance

Calculating PEEP:
If we do an expiratory hold it brings the flow and volume to zero. This removes resistance and alveolar compliance.
Pmusc + Pvent = R x F + V/C + PEEP
↓
Pmusc + Pvent = R x (0) + (0)/C + PEEP
↓
Pexp = PEEP
Figure 10: Pressure Scalar and PEEP

If you assume the previous breath was completely exhaled, Pexp should equal the PEEP on the ventilator. If the Pexp is higher than the set PEEP this would imply an incomplete expiration as seen with air-trapping, auto-peep, and vent dyssynchrony. A Pexp lower than expected would imply a leak.
The equation of motion can be intimidating with all the variables, but at its core it is simple. In order to get gas flow in and out of the lung, it requires pressure. The pressure is needed to overcome the resistance and then needed to expand the alveoli. Hopefully, this helps show the simplicity.
Total Pressure = Resistive Pressure + Distending Pressure
Pmusc + Pvent = R x F + V/C + PEEP

Figure 11: Pressure scalar with inspiratory hold

Figure 12: Pressure scalar without inspiratory hold

References:
UBC Critical Care. Mechanics of Ventilation. http://www.ubccriticalcaremedicine.ca/rotating/material/Lecture_1%20for%20Residents.pdf accessed 4/7/21
Thoracic Key. How a breath is delivered. 2016. https://thoracickey.com/how-a-breath-is-delivered/ accessed 4/7/21
Thoracic Key. Mechanical Ventilators. 2016 https://thoracickey.com/mechanical-ventilators/ accessed 4/7/21
Nandyala S. Basics of Mechanical Ventilation. 2017 https://pocketicu.com/index.php/2017/02/24/mechanical-ventilation/#:~:text=Ventilator%20can%20control%20only%20one,resistance%20and%20v%CC%87%20is%20flow. accessed 4/7/21
Kenny JE. ICU Physiology in 1000 Words: The Mechanical Power & Ventilator-Induced Lung Injury.2019. https://pulmccm.org/review-articles/icu-physiology-in-1000-words-the-mechanical-power-ventilator-induced-lung-injury/ accessed 4/7/21