Intravascular Volume
Table 1: Body Fluid Compartments: Where is the fluid?

Figure 1: Body Fluid Compartments
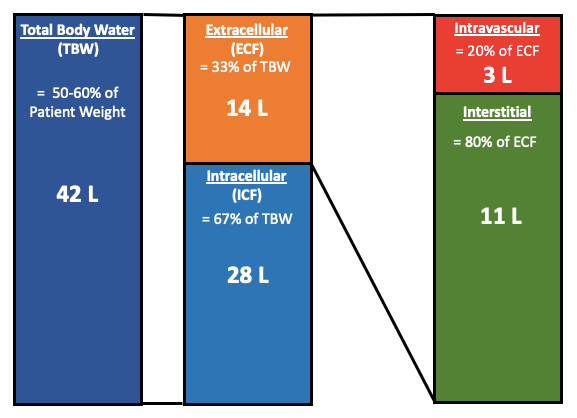
The amount of fluid in the intravascular space is only 3 liters, keep this in mind when giving patients fluids.
What Controls Intravascular Volume:
​
Intravascular volume is controlled by Starling forces. Starling forces are the pressures that want to drive fluid into the intravascular space and the pressures that want to drive fluid out of the intravascular space.
Starling Forces:
1. Oncotic Pressure
2. Osmotic Pressure
3. Hydrostatic Pressure
Starling equation:
The Starling equation determines the rate of fluid either in or out of the intravascular space. This is done at the level of the capillaries. Jv is the rate at which it moves in volume per sec (m3/s). It is the difference between the hydrostatic forces minus the difference in oncotic forces.
Jv = LpS [(Pc - Pi] - σ(πc-πi)]
Jv = Rate of fluid entering or leaving the intravascular space
Lp = Hydraulic permeability coefficient
S = Surface area of membrane
Pc = Capillary hydrostatic pressure
Pi = Interstitial hydrostatic pressure (small)
πc = Capillary oncotic pressure
πi = Interstitial oncotic pressure (negligible)
σ = reflection coefficient for protein
​
At equilibrium, the fluid is pushed out of the intravascular space at the capillary level because the Starling equation becomes positive, and then the fluid comes back in to the intravascular space at the venule level as the Starling equation becomes negative.
By understanding this equation it is easy to see that in order to keep fluid in the intravascular space the capillary oncotic pressure must be increased or the capillary hydrostatic pressure decreased.
​
​
​
​
​
​
​
​
​
​
​
Osmotic pressure:
Osmotic pressure occurs in the intravascular and interstitial compartments and opposes each other. The osmolarity in the intravascular space wants to pull fluid in, the same is true for the osmolarity in the interstitial space, but it wants to pull fluid into the interstitial space.
​
The electrolytes completely dissociate into ions and are major contributors to the osmolarity of the interstitial and intravascular space. In fact, 95% of the osmolarity in the intravascular space is electrolytes. The reason that they do not contribute to the Starling equation is that electrolytes can cross the capillary membrane and, between diffusion and osmosis, the osmolarity equilibrates between the two spaces making the net movement due to electrolytes zero.
The van ‘t Hoff equation shows what determines the osmotic pressure
Osmotic pressure = π = iMRT
i = number of ions a solute will form when dissolved in water (van’t Hoff factor)
M = Molar concentration of the solution (mol/L)
R = Ideal gas constant (0.08206 L atm/mol K)
T = Temperature in Kelvin (K)
Again, since the electrolytes can diffuse across to the interstitial space, they normally do not participate much and the main determinate for keeping fluid in the intravascular space is oncotic pressure.
So why is osmotic pressure shown in figure 2?
The reason that osmotic pressure is represented in the forces for fluid movement is to represent opportunities for clinical intervention. This post is more about clinical opportunities for intervention more than most because physiologically electrolytes do not do much at baseline. But clinically, the osmolarity can be changed and used for resuscitation.
The logical next step in keeping volume intravascularly is giving hypertonic solutions. This is when a solution is given where the osmolarity is higher than the serum osmolarity. hypertonic solutions will change the equilibrium and, through osmosis, will drive fluid from the interstitial space to the intravascular space.
​
The change in electrolyte concentration will also cause fluid movement out of the intracellular space as well.
Figure 2: Starling Forces

Figure 3: Osmosis

Oncotic pressure:
The intravascular space has proteins that also help contribute to the osmotic pressure, although it can be looked at as its own thing since it is protein. There are significantly fewer proteins in the interstitial space and can be considered zero.
​
Albumin works better clinically than anticipated, this phenomenon is due to the Gibbs-Donnan Effect.
​
Gibbs-Donnan Effect:
Albumin is negatively charged. This causes a change in the osmolarity gradient between the interstitial and intravascular space. Cations are more inclined to stay in the intravascular space (ratio 100:95) and anions in the interstitial space (ratio 100:105) which is shown in Table 1.
Table 2: Gibbs-Donnan Effect on Osmolarity

A normal plasma protein level of 0.9mmol/L should generate an oncotic pressure of 17.4mmHg (1mmol/L = 19.3mmHg), but actually generates an oncotic pressure of 25mmHg. Due to the Gibbs-Donnan effect attracting cations to its negative charge it adds 0.4mmol/L to the overall concentration, creating 1.3mmol/L, which accounts for the oncotic pressure increase from 17.4mmHg to 25mmHg. The oncotic pressure will pull water into the intravascular space and the Gibbs-Donnan effect increases the oncotic pressure and total water being pulled in. This is why a normal albumin level is so important to the body’s fluid equilibrium (See Figure 1). When the protein level drops, which can be seen in critical illness, dilutional resuscitation, and malnutrition, the oncotic pressure and Gibbs-Donnan effect are lost. This leads to third-spacing into the interstitial space with intravascular depletion. Physiologically, once the oncotic pressure drops below 11 mmHg the benefits are lost and spontaneous third-spacing occurs. Clinically, this loss of oncotic pressure benefit is around 2g/dL.
Figure 4: The Gibbs-Donnan Effect on Intravascular Fluid

Hydrostatic pressure:
The 3rd force, hydrostatic pressure, is the pressure that wants to push fluid out of the space. The intravascular hydrostatic pressure wants to push fluid into the interstitial space and the interstitial hydrostatic pressure wants to push fluid into the intravascular space. The interstitial hydrostatic pressure is low and usually negligible.
Resources:
-
Blogs/Podcasts/Videos:
-
Lectures/Handouts:
-
References:
-
Tobias A, Ballard BD, Mohiuddin SS. Physiology, Water Balance. [Updated 2020 Oct 7]. In: StatPearls [Internet]. Treasure Island (FL): StatPearls Publishing; 2021 Jan-. Available from: https://www.ncbi.nlm.nih.gov/books/NBK541059/
-
Starling forces and fluid exchange in the microcirculation: Deranged Physiology. https://derangedphysiology.com/main/cicm-primary-exam/required-reading/cardiovascular-system/Chapter%20471/starling-forces-and-fluid-exchange-microcirculation. Accessed 7/12/21.
-
Nguyen MK, Kurtz I. Determinants of plasma water sodium concentration as reflected in the Edelman equation: role of osmotic and Gibbs-Donnan equilibrium. American Journal of Physiology-Renal Physiology. 2004;286(5):F828-F37.
-
Yartsef A 2015;Pageshttps://derangedphysiology.com/main/cicm-primary-exam/required-reading/cellular-physiology/Chapter%20121/gibbs-donnan-effect on 12/1/20.
-
Yartsef A. 2020; Pages https://derangedphysiology.com/main/cicm-primary-exam/required-reading/body-fluids-and-electrolytes/manipulation-fluids-and-electrolytes/Chapter%20013/osmotic-pressure-and-oncotic-pressure on 7/22/21.
-
Hahn RG, Lyons G. The half-life of infusion fluids: An educational review. Eur J Anaesthesiol. 2016;33(7):475-482. doi:10.1097/EJA.0000000000000436
-
5 - Disturbances of Free Water, Electrolytes, Acid-Base Balance, and Oncotic Pressure, Editor(s): Peter D. Constable, Kenneth W. Hinchcliff, Stanley H. Done, Walter Grünberg, Veterinary Medicine (Eleventh Edition), W.B. Saunders, 2017, Pages 113-152, ISBN 9780702052460, https://doi.org/10.1016/B978-0-7020-5246-0.00005-X.
-
Finfer S, Bellomo R, Boyce N, et al. A comparison of albumin and saline for fluid resuscitation in the intensive care unit. N Engl J Med. 2004;350(22):2247-2256. doi:10.1056/NEJMoa040232
-
Caironi P, Tognoni G, Masson S, et al. Albumin replacement in patients with severe sepsis or septic shock. N Engl J Med. 2014;370(15):1412-1421. doi:10.1056/NEJMoa1305727
-
Mårtensson J, Bihari S, Bannard-Smith J, et al. Small volume resuscitation with 20% albumin in intensive care: physiological effects : The SWIPE randomised clinical trial. Intensive Care Med. 2018;44(11):1797-1806. doi:10.1007/s00134-018-5253-2
-